Topics
2020.3.5
Flying strong magnetic field devices change experiments
High magnetic field condensed matter physics group
What do you imagine with a strong magnetic field? If a levitating Chikuwa comes into your mind, you’re the one who watched TV the other day and was “scorched by Chico”. Aside from that, in the first place, a strong magnetic field is not a special field of studying the magnetic field itself, but a field of studying materials using a magnetic field. A magnetic field is a field created by the movement of charges. Conversely, when a magnetic field is applied, it affects the movement of charges and the spin of electrons, so it is used in various fields in physics, such as magnetism, superconductivity, and semiconductors. Another important area is measurement system such as MRI taking an image of a living body using a magnetic field.
There are few ways to create a magnetic field: using a superconducting magnet without electrical resistance, cooling a resistive magnet with water and applying a current to obtain a DC magnetic field, and instantaneously discharging the current stored in a capacitor or the like. The third one-a pulsed magnetic field provides a short but strong magnetic field. A pulse magnetic field has the advantage that the power supply and cooling are much smaller than DC fields. However, usually, strong magnetic field devices are large and so users have to come to a strong magnetic field facility to conduct experiments. However, there are experiments that cannot be done in this way such as synchrotron radiation, free electron lasers, and neutrons. We have developed a mobile strong magnetic field generator that has made this possible.
Fig. 1 is a photograph of an experiment with the X-ray free electron laser SACLA. The power supply of the magnetic field generator is slightly larger than the compressor of the refrigerator, and fits in a basket similar to a so-called moving single pack. Fig. 2 shows a split coil for diffraction experiments, which is only 25-30 mm in size, but can generate a very strong magnetic field of 40 T. If you fly with it by an airplane, you can conduct experiments at overseas facilities. One of the advantages of such a small device is that the leakage magnetic field can be ignored. For neutrons and X-rays, shielding and diffractometers are made of iron, so it is necessary to change to special specifications to introduce superconducting magnets. Our small pulse magnets don’t need to do that, they can be easily attached to existing equipment, and there is no barrier to start.
Fig. 1
Fig. 2
Here are two recent examples of what kind of experiments are possible. Fig. 3 is an experiment of the magnetic field induced charge density wave of the high-temperature superconductor YBa2Cu3O7-d [1]. In this system, when a magnetic field is applied to weaken the superconductivity, the charge order of incommensurate wave vector appears, which is very interesting. However, ordinary superconducting magnets cannot completely suppress superconductivity, which is where pulsed magnetic fields come into play. In this experiment, a weak signal of 6 orders of magnitude less from the fundamental Bragg peak was observed in a single pulse magnetic field of 40 T using the X-ray free electron laser of Stanford laboratory, and the phase diagram of the charge density wave in the low hole concentration region was completed.
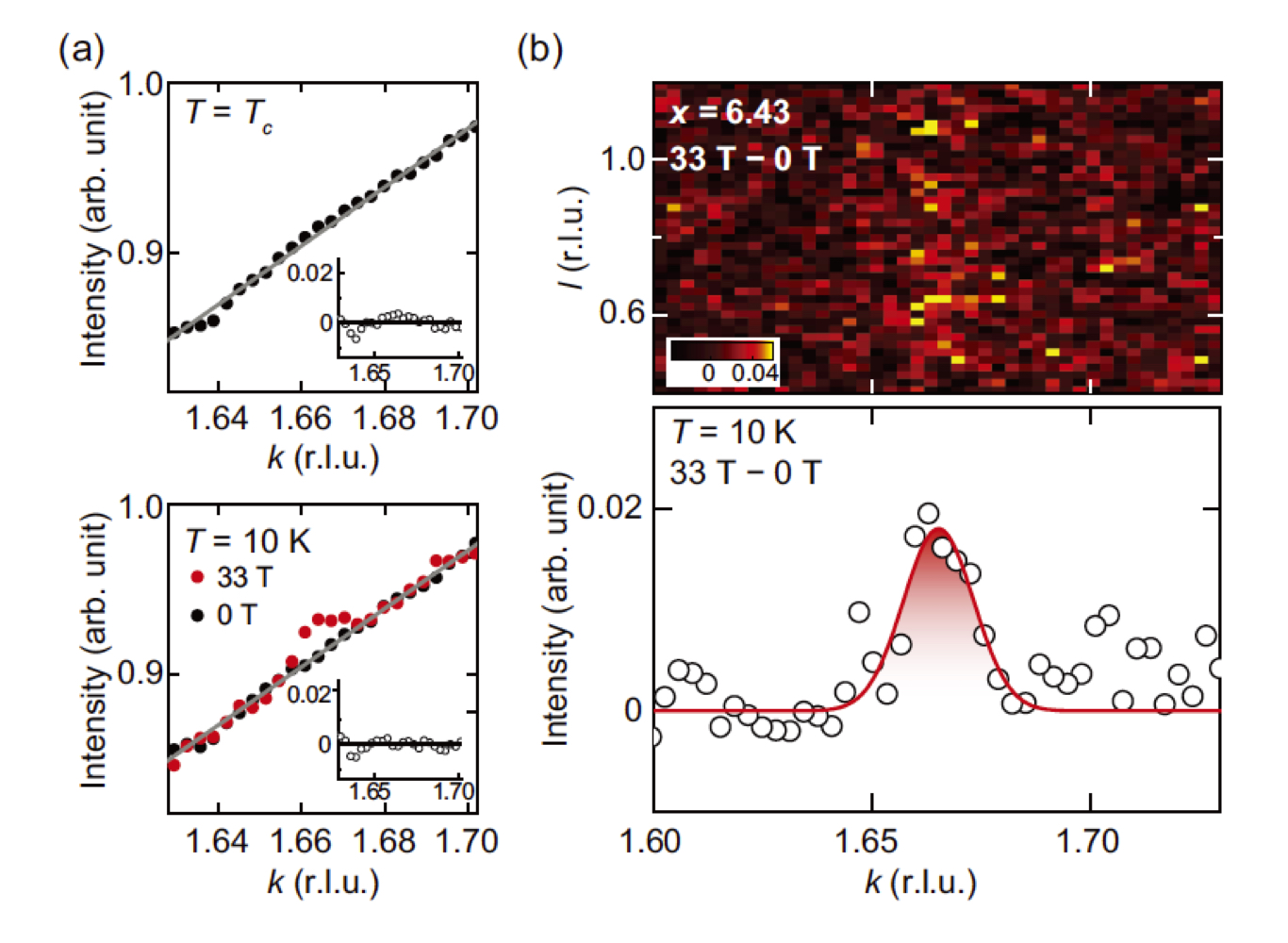
Fig. 3
The second experiment is neutron diffraction. Fig. 4 shows the diffraction pattern of the multiferroic material LiNiPO4 by a white beam Laue method in a pulse magnetic field of 40.5 T. It was measured in a pulse magnetic field using pulsed neutrons of J-PARC [2]. In white neutrons, one uses the time-of-flight (TOF) method, which is related to neutron energy and wavelength. TOF can be converted in proportion to wave number. The different colored peaks correspond to different magnetic field regions as shown on the vertical axis, indicating that the magnetic wave number changes in the changing magnetic field. In this material, there are six successive phase transitions in magnetic fields up to 55 T, and the ferroelectric phase and the non-ferroelectric phase are switched. To understand this, it was necessary to determine the magnetic structure in a magnetic field. In this experiment, it was found that the system is ferroelectric if it was a commensurate phase, and non-ferroelectric if it was incommensurate. Possible models can be screened by this unique information. As such, these experiments are being conducted at various facilities in Japan, Switzerland, France, Germany, and so on, and our strong magnetic field devices are flying today. And, of course, ourselves.
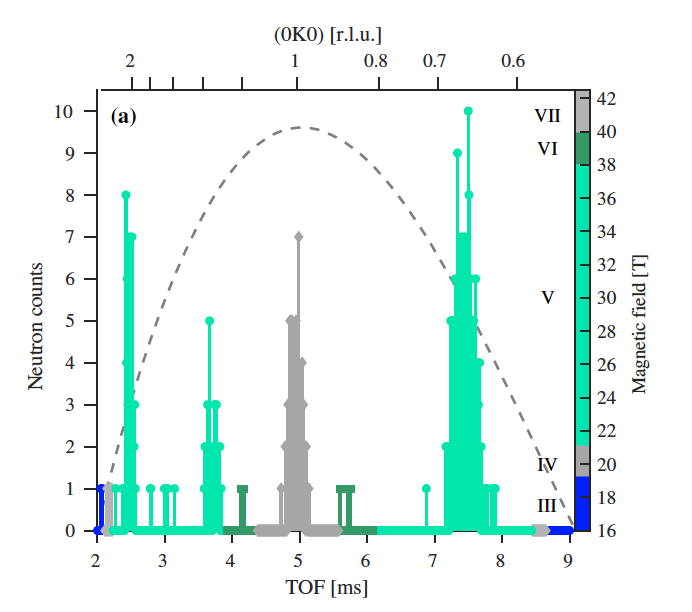
Fig. 4
References
[1] Phys. Rev. B 97(2018), 224513.
[2] Phys. Rev. B 101(2020) 024403.